The tech giant poached several top Google researchers to help build a powerful AI tool that can diagnose patients and potentially cut health care costs.
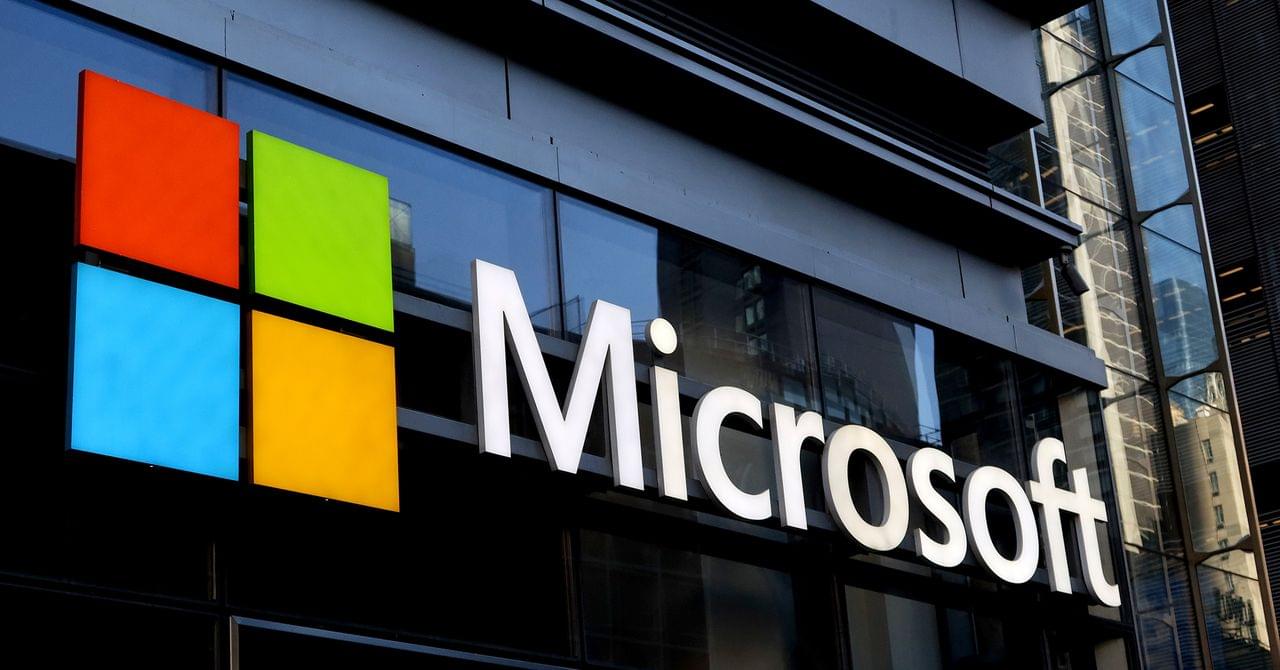
In radiation therapy, precision can save lives. Oncologists must carefully map the size and location of a tumor before delivering high-dose radiation to destroy cancer cells while sparing healthy tissue. But this process, called tumor segmentation, is still done manually, takes time, varies between doctors—and can lead to critical tumor areas being overlooked.
Now, a team of Northwestern Medicine scientists has developed an AI tool called iSeg that not only matches doctors in accurately outlining lung tumors on CT scans but can also identify areas that some doctors may miss, reports a large new study.
Unlike earlier AI tools that focused on static images, iSeg is the first 3D deep learning tool shown to segment tumors as they move with each breath—a critical factor in planning radiation treatment, which half of all cancer patients in the U.S. receive during their illness.
New research from the University of Pittsburgh School of Medicine and La Jolla Institute for Immunology, published today in Nature Microbiology, reveals an opportunity for developing a therapy against cytomegalovirus (CMV), the leading infectious cause of birth defects in the United States.
Researchers discovered a previously unappreciated mechanism by which CMV, a herpes virus that infects the majority of the world’s adult population, enters cells that line the blood vessels and contributes to vascular disease. In addition to using molecular machinery that is shared by all herpes viruses, CMV employs another molecular “key” that allows the virus to sneak through a side door and evade the body’s natural immune defenses.
The finding might explain why efforts to develop prophylactic treatments against CMV have, so far, been unsuccessful. This research also highlights a new potential avenue for the development of future antiviral drugs and suggests that other viruses of the herpes family, such as Epstein-Barr and chickenpox, could use similar molecular structures to spread from one infected cell to the next while avoiding immune detection.
This study reports on self-aggregating injectable microcrystals for administering long-acting drug implants via low-profile needles, a key factor in patient adoption. Microcrystal self-aggregation is engineered through a solvent exchange process to form depots with minimal polymer excipient, demonstrating enhanced long-term release of a model contraceptive drug in rodents.
ImageimagePoorly energized mitochondria trap a subpopulation of mitochondrial precursor proteins in the intermembrane space. This article introduces ‘mitochondrial triage of precursor proteins’ (MitoTraP) as a mechanism that prevents the mistargeting of non-imported proteins to the nucleus and reduces proteotoxic effects.
Miscarriage denotes the loss of a pregnancy before viability (1). Approximately one in three embryos perishes following implantation in healthy women, although often before routine detection of pregnancy (2, 3). This attrition rate reflects the high prevalence of chromosomal errors in preimplantation human embryos (4) and the physiological role of the endometrium in selecting against low-fitness embryos (2, 3, 5, 6). The pooled miscarriage risk in all clinically recognized pregnancies is an estimated 15% (1), with most losses (~90%) occurring before the onset of uteroplacental perfusion at the end of the first trimester (7). Epidemiological studies consistently highlight that two factors, maternal age and the number of preceding pregnancy losses, disproportionally affect miscarriage rates (7–9). The age-dependent risk reflects the increase in aneuploid pregnancies in women aged 35 years and older, mirroring the incidence of meiotic chromosome errors in oocytes and embryos (10, 11). Each prior pregnancy loss further compounds the risk stepwise by 5 to 10% (7–9), but the underlying mechanism is unknown. A plausible but untested hypothesis is that the recurrence risk of miscarriage reflects the frequency of menstrual cycles culminating in an endometrial environment permissive of embryo implantation but inadequately prepared for decidual transformation (3), that is, the formation of a robust immunotolerant matrix that anchors and supports the semiallogenic placenta throughout pregnancy (2, 5).
Each menstrual cycle starts with the shedding of the superficial endometrial layer, bleeding, and reepithelization of the basal layer. Following menstruation, estradiol-dependent regeneration of the superficial layer, on average, quadruples the thickness and volume of the uterine mucosa before ovulation (12). Local morphogen and cytokine gradients regulate epithelial and stromal cell proliferation, resulting in tissue stratification and positional cell specification. After ovulation, progesterone acting on this spatial template triggers a decidual reaction, an endogenous inflammatory tissue response that heralds the start of the 4-day midluteal implantation window (2, 13). Histologically, the implantation window coincides with the onset of glandular secretion, marked oedema, and proliferative expansion and differentiation of uterine natural killer (uNK) cells (14, 15).
Cancer cells turbocharge themselves by stealing the energy-producing units from neurons in tumours, scientists report today in Nature 1. This act of thievery seems to give cancer cells a boost to help them survive when they metastasize, or spread to distant organs.
The findings show that cancer cells siphon off neurons’ mitochondria — organelles that generate most of a cell’s energy — through ultrathin tubes that grow between the two types of cell. The purloined mitochondria increase cancer cells’ ability to withstand the stress of shooting through blood vessels during metastasis.
“Now we have a new culprit for metastasis, which means we have a new target to block metastasis,” says study co-author Simon Grelet, a cancer neurobiologist at the University of South Alabama in Mobile. “And metastasis is what make cancers so deadly.”
The theft probably helps the cells to spread around the body, and preventing it could provide a path to treatment, researchers say.