Did Mars once have life as we know it deep in its ancient past? A recent study published in Proceedings of the National Academy of Sciences might be one st | Space
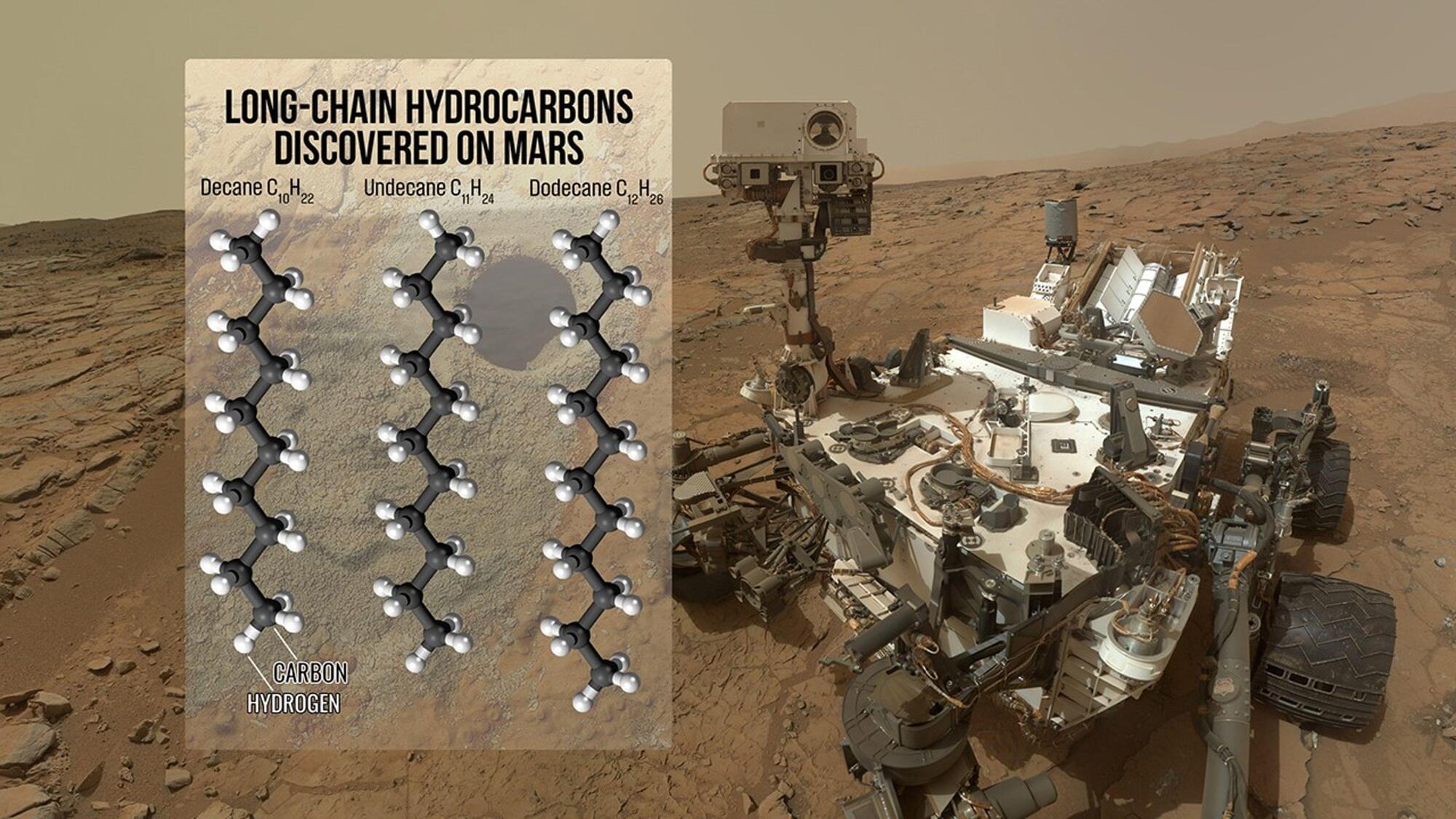
The heating effect of microwaves has long been used to accelerate reactions. A new experiment shows that microwaves can also excite molecules into a less reactive state.
According to Arrhenius’ law, heating increases the energy of molecules so that more of them can overcome the activation barrier and undergo a chemical reaction. One way to deliver heat is via microwave radiation. Since its early use in chemical synthesis, scientists have noticed that microwave-induced reactions often proceed differently compared with ones enhanced with oil baths and other traditional heating methods. This finding has led to ongoing speculation and debate—and even controversy—about the existence of microwave effects beyond heating [1]. Now Valentina Zhelyazkova of the Swiss Federal Institute of Technology (ETH) Zurich and her collaborators have demonstrated that microwaves can both speed up and slow down chemical reactions [2]. The discovery provides clear evidence of the nonthermal influence of microwaves on chemical processes. It also opens a path toward controlling reactions and understanding them more deeply.
In their investigation Zhelyazkova and her collaborators manipulated the rate of the gas-phase reaction between positively charged helium ions (He+) and carbon monoxide (CO) molecules: He++ CO → He + C++ O. According to so-called capture theory, the reaction’s rate depends on the rotational states of CO, whose quantized energies lie within the microwave band (Fig. 1). The experiment began with the preparation of separate supersonic beams of He atoms and CO molecules via high-pressure expansion into vacuum. The CO molecules were initially in the rotational ground state. By applying a precisely timed microwave pulse before the reaction, the researchers excited a fraction of the population to the first rotationally excited state, which is less reactive than the ground state. The fraction that was excited could be fine-tuned by changing the duration of the microwave pulse.
As the world makes more use of renewable energy sources, new battery technology is needed to store electricity for the times when the sun isn’t shining, and the wind isn’t blowing.
“Current lithium batteries have reached their limitations in terms of energy storage capability, life cycle, and safety,” says Xiaolei Wang, a professor of chemical engineering at the University of Alberta in Edmonton. “They’re good for applications like electric vehicles and portable electronics, but they’re not suitable for large-scale grid-level energy storage.”
With the help of the Canadian Light Source at the University of Saskatchewan, Wang and his team are developing new technologies to help make grid-level aqueous batteries that can use seawater as an electrolyte. The study is published in the journal Advanced Materials.
Researchers have developed a new material that, by harnessing the power of sunlight, can clear water of dangerous pollutants. Created through a combination of soft chemistry gels and electrospinning—a technique where electrical force is applied to liquid to craft small fibers—the team constructed thin fiber-like strips of titanium dioxide (TiO₂), a compound often utilized in solar cells, gas sensors and various self-cleaning technologies.
Despite being a great alternative energy source, solar fuel systems that utilize TiO₂ nanoparticles are often power-limited because they can only undergo photocatalysis, or create chemical reactions, by absorbing non-visible UV light. This can cause significant challenges to implementation, including low efficiency and the need for complex filtration systems.
Yet when researchers added copper to the material to improve this process, their new structures, called nanomats, were able to absorb enough light energy to break down harmful pollutants in air and water, said Pelagia-Iren Gouma, lead author of the study and a professor of materials science and engineering at The Ohio State University.
A research team led by Professor Hyung-Joon Shin from the Department of Materials Science and Engineering at UNIST has succeeded in elucidating the quantum phenomenon occurring within a triangular cluster of three water molecules. The work is published in the journal Nano Letters.
Their findings demonstrate that the collective rotational motion of water molecules enhances proton tunneling, a quantum mechanical effect where protons (H+) bypass energy barriers instead of overcoming them. This phenomenon has implications for chemical reaction rates and the stability of biomolecules such as DNA.
The study reveals that when the rotational motion of water molecules is activated, the distances between the molecules adjust, resulting in increased cooperativity and facilitating proton tunneling. This process allows the three protons from the water molecules to collectively surmount the energy barrier.
In recent years, many engineers have been trying to develop hardware components that could emulate the functions of various biological systems, including synapses, the human skin and nerves. These bio-inspired systems include what are referred to as artificial nerves, systems designed to emulate the role of nerves in the body of humans and other animals.
Artificial nerves could be useful for a wide range of applications, ranging from systems for repairing damaged nerves to brain-computer interfaces, highly precise sensors and other advanced electronics. So far, however, the engineering of nerve-inspired systems that operate at biologically compatible frequencies and realistically replicate the function of nerves has proved challenging.
Researchers at Xi’an Jiaotong University in China and Technical University of Munich recently developed a new high-frequency artificial nerve with a unique design that optimizes the transport of ions and electrons, while also rapidly responding to signals and retaining charge-related information. This nerve-inspired system, introduced in a paper published in Nature Electronics, is based on homogenously integrated organic electrochemical transistors.
Astronomers uncover extremely hot and violent eruption from first ever near-infrared analysis of a recurrent nova outside of the Milky Way Galaxy. Using the Gemini South telescope, one half of the International Gemini Observatory, partly funded by the U.S. National Science Foundation and operated by NSF NOIRLab, and the Magellan Baade Telescope, astronomers have for the first time observed a recurring nova outside of the Milky Way in near-infrared light. The data revealed highly unusual chemical emissions as well as one of the hottest temperatures ever reported for a nova, both indicative of an extremely violent eruption.
Nova explosions occur in binary star systems in which a white dwarf — the dense remnant of a dead star — continually siphons stellar material from a nearby companion star. As the outer atmosphere of the companion gathers onto the surface of the white dwarf it reaches temperatures hot enough to spark an eruption.
Almost all novae discovered to-date have been observed to erupt only once. But a few have been observed to erupt more than once, and are classified as recurrent novae. The span between eruptions for these novae can vary from as little as one year to many decades [1].
A new method inspired by coral reefs can capture carbon dioxide from the atmosphere and transform it into durable, fire-resistant building materials, offering a promising solution for carbon-negative construction.
The approach, developed by USC researchers and detailed in a study published in npj Advanced Manufacturing, draws inspiration from the ocean’s coral reefs’ natural ability to create robust structures by sequestering carbon dioxide. The resulting mineral-polymer composites demonstrate extraordinary mechanical strength, fracture toughness and fire-resistance capabilities.
“This is a pivotal step in the evolution of converting carbon dioxide,” said Qiming Wang, associate professor of civil and environmental engineering at the USC Viterbi School of Engineering. “Unlike traditional carbon capture technologies that focus on storing carbon dioxide or converting it into liquid substances, we found this new electrochemical manufacturing process converts the chemical compound into calcium carbonate minerals in 3D-printed polymer scaffolds.”
Researchers at Indiana University and Wuhan University in China have unveiled a groundbreaking chemical process that could streamline the development of pharmaceutical compounds, chemical building blocks that influence how drugs interact with the body. Their study, published in Chem, describes a novel light-driven reaction that efficiently produces tetrahydroisoquinolines, a group of chemicals that play a crucial role in medicinal chemistry.
Tetrahydroisoquinolines serve as the foundation for treatments targeting Parkinson’s disease, cancer, and cardiovascular disorders. These compounds are commonly found in medications such as painkillers and drugs for high blood pressure, as well as in natural sources like certain plants and marine organisms.
Traditionally, chemists have relied on well-established but limiting methods to synthesize these molecules. The new research, co-authored by Kevin Brown, the James F. Jackson Professor of Chemistry in the College of Arts and Sciences at Indiana University Bloomington, and Professors Xiaotian Qi, Wang Wang, and Bodi Zhao of Wuhan University, presents a fundamentally different approach.