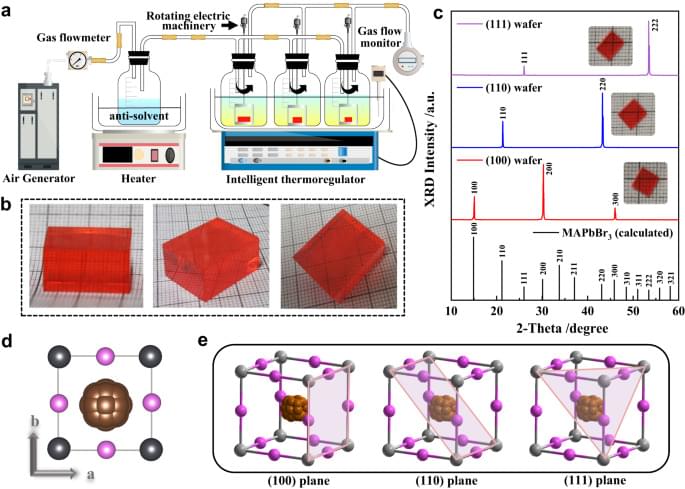
Based on the differently oriented MAPbBr3 single-crystal wafers, this study presents innovative findings primarily in two aspects. First, utilizing polarization-dependent ultrafast time-resolved spectroscopy, the anisotropic dynamics of photoexcited carriers on the picosecond timescale was first revealed. This discovery provides a deeper understanding of the ultrafast carrier relaxation pathways from the perspective of crystal orientation. It holds significant implications for exploring and expanding the potential of perovskite single crystals in polarization-sensitive and ultrafast optoelectronics applications, such as optical modulators, high-speed light polarization sensors, and ballistic transistors, which require both polarization sensitivity and high-field running capability simultaneously. However, a comprehensive understanding to correlate the observed polarization-dependent dynamics with the crystallographic structures has not been achieved yet due to limitations in current excited-state experimental techniques. Further progress will rely on employing more advanced ultrafast probing techniques, in combination with theoretical simulations, to comprehensively elucidate the observed carrier dynamics behind the underlying excited structure.
Second, by employing femtosecond laser processing, luminescent patterns with a remarkable three-order-of-magnitude PL enhancement on the bulk single crystals were achieved. The observed enhancement can be ultimately attributed to the synergy of three factors: the limited carrier diffusion length, the increase in shallow trap-assisted recombination centers, and the passivation of deep traps within the femtosecond laser-induced tentacle-like microstructures. In addition to offering a convenient top-down strategy for enhancing the photoluminescence intensity of bulk crystals, this study has also provided an in-depth understanding of the luminescence mechanism from multiple spatial (bulk and micro/nanoscale) and temporal (steady and transient-state) dimensions.