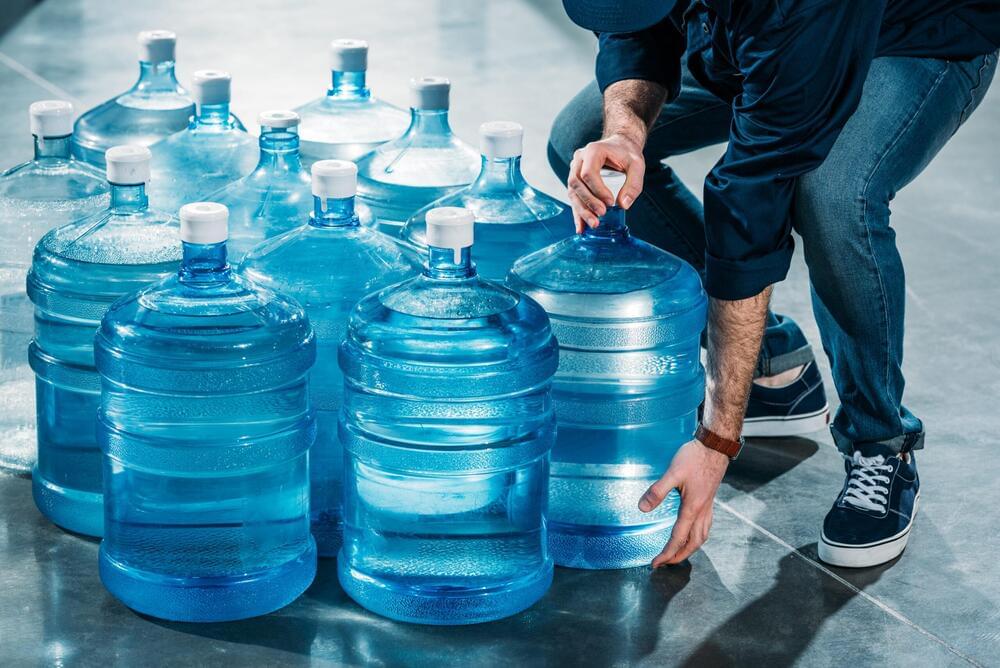
Earth’s H2O is 97 percent seawater, and most of the remaining 3 percent is inaccessible, frozen in glaciers or permafrost. Only a small portion, about half of a percent, exists as freshwater in aquifers and rivers that humans can tap into. A process called, however, allows us to dip into the oceans to satisfy our thirst.
Desal has been around for decades and is used to make both seawater and salty groundwater drinkable. But scientists think that it will become increasingly important in a warmer, drier future. In a recent UN-led review, researchers stated that “‘conventional’ sources of water such as rainfall, snowmelt and river runoff captured in lakes, rivers, and aquifers are no longer sufficient to meet human demands in water-scarce areas.”
During a media roundtable at the 2019 American Geophysical Union conference, Peter Fiske, director of the Water-Energy Resilience Research Institute at Lawrence Berkeley National Laboratory, discussed why we might need to more strongly consider this technology—at times written off for its high costs and energy use—to stabilize water supplies in the future. Here’s what you need to know.