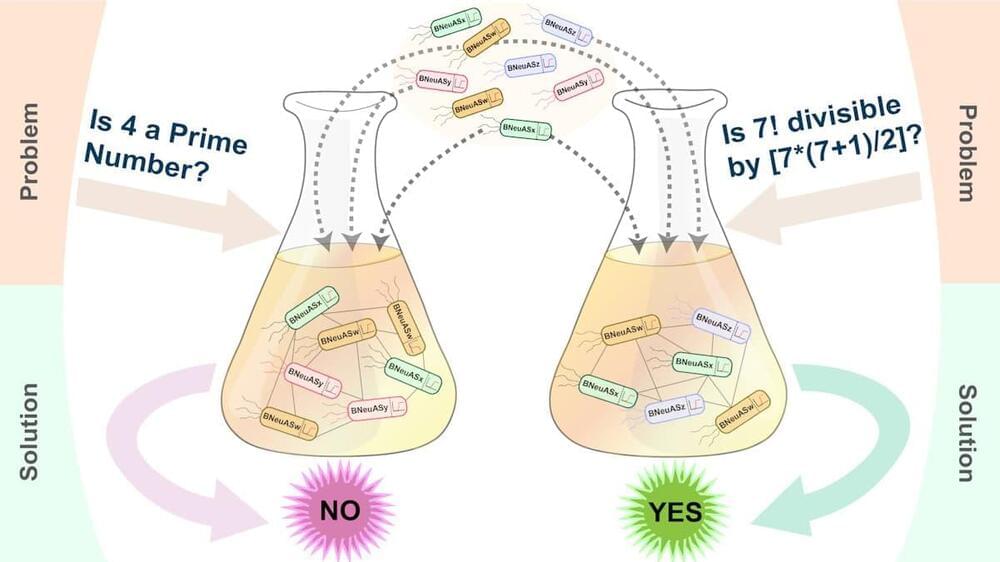
Researchers have developed a groundbreaking system that uses bacteria to mimic the problem-solving capabilities of artificial neural networks.
Cell-based biocomputing is a novel technique that uses cellular processes to perform computations. Such micron-scale biocomputers could overcome many of the energy, cost and technological limitations of conventional microprocessor-based computers, but the technology is still very much in its infancy. One of the key challenges is the creation of cell-based systems that can solve complex computational problems.
Now a research team from the Saha Institute of Nuclear Physics in India has used genetically modified bacteria to create a cell-based biocomputer with problem-solving capabilities. The researchers created 14 engineered bacterial cells, each of which functioned as a modular and configurable system. They demonstrated that by mixing and matching appropriate modules, the resulting multicellular system could solve nine yes/no computational decision problems and one optimization problem.
The cellular system, described in Nature Chemical Biology, can identify prime numbers, check whether a given letter is a vowel, and even determine the maximum number of pizza or pie slices obtained from a specific number of straight cuts. Here, senior author Sangram Bagh explains the study’s aims and findings.