Eco-friendly advancements promise a cleaner, greener approach to producing essential materials.
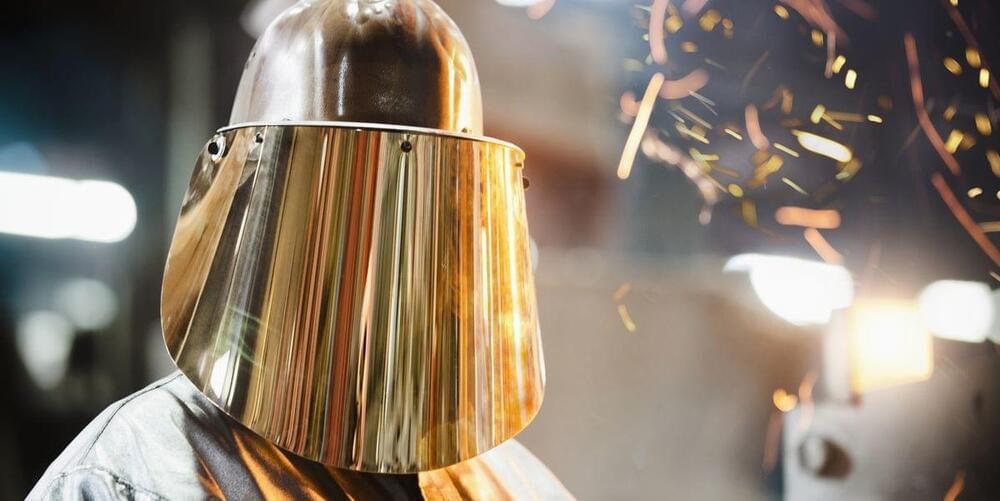
However, if long and thin strips of graphene (termed graphene nanoribbons) are cut out of a wide graphene sheet, the quantum charge carriers become confined within the narrow dimension, which makes them semi-conducting and enables their use in quantum switching devices. As of today, there are a number of barriers to using graphene nanoribbons in devices, among them is the challenge of reproducibly growing narrow and long sheets that are isolated from the environment.
In this new study, the researchers were able to develop a method to catalytically grow narrow, long, and reproducible graphene nanoribbons directly within insulating hexagonal boron-nitride stacks, as well as demonstrate peak performance in quantum switching devices based on the newly-grown ribbons. The unique growth mechanism was revealed using advanced molecular dynamics simulation tools that were developed and implemented by the Israeli teams.
These calculations showed that ultra-low friction in certain growth directions within the boron-nitride crystal dictates the reproducibility of the structure of the ribbon, allowing it to grow to unprecedented lengths directly within a clean and isolated environment.
A team led by Chen Xianhui and Professor Xiang Ziji from the CAS Key Laboratory of Strongly-Coupled Quantum Matter Physics and the Department of Physics at the University of Science and Technology of China, uncovered a unique superconducting state characterized by one-dimensional superconducting stripes. This state is induced by the ferromagnetic proximity effect in an oxide heterostructure made up of ferromagnetic EuO and (110)-oriented KTaO3 (KTO). Their findings were published in Nature Physics.
The academic community concurs that the emergence of unconventional superconducting pairings is intricately linked to magnetism, particularly in copper oxides and iron-based high-temperature superconductors. Magnetic fluctuations are deemed pivotal in the genesis of high-temperature superconductivity, where the interplay between superconductivity and magnetism gives rise to superconducting states exhibiting unique spatial modulation. Superconducting oxide heterostructures encompassing magnetic structural units emerge as an optimal platform for investigating such superconducting states.
Building upon their prior achievements, the research team delved deeper into the superconductivity of this system and its relationship with the ferromagnetic proximity effect, meticulously adjusting the carrier concentration of the two-dimensional electron gas residing at the interface. They uncovered an intriguing in-plane anisotropy in superconductivity among samples with low carrier concentrations, which nevertheless vanished in samples exhibiting higher carrier concentrations.
The NASA/ESA/CSA James Webb Space Telescope has captured the sharpest infrared images to date of one of the most distinctive objects in our skies, the Horsehead Nebula. These observations show a part of the iconic nebula in a whole new light, capturing its complexity with unprecedented spatial resolution.
Webb’s new images show part of the sky in the constellation Orion (The Hunter), in the western side of the Orion B molecular cloud. Rising from turbulent waves of dust and gas is the Horsehead Nebula, otherwise known as Barnard 33, which resides roughly 1,300 light-years away.
The nebula formed from a collapsing interstellar cloud of material, and glows because it is illuminated by a nearby hot star. The gas clouds surrounding the Horsehead have already dissipated, but the jutting pillar is made of thick clumps of material that is harder to erode. Astronomers estimate that the Horsehead has about 5 million years left before it too disintegrates. Webb’s new view focuses on the illuminated edge of the top of the nebula’s distinctive dust and gas structure.
“Mathematics, rightly viewed, possesses not only truth, but supreme beauty — a beauty cold and austere, like that of sculpture.”
- Bertrand Russell (1972 — 1970) A History of Western Philosophy
https://mathshistory.st-andrews.ac.uk/Biographies/Russell/
The book was written during the Second World War, having its origins in a series of lectures on the history of philosophy that Russell gave at the Barnes Foundation in Philadelphia during 1941 and 1942.[2] Much of the historical research was done by Russell’s third wife Patricia. In 1943, Russell received an advance of $3000 from the publishers, and between 1944 and 1945 he wrote the book while living at Bryn Mawr College. The book was published in 1946 in the United Kingdom and a year later in the US. It was re-set as a ‘new edition’ in 1961, but no new material was added. Corrections and minor revisions were made to printings of the British first edition and for 1961’s new edition; no corrections seem to have been transferred to the American edition (even Spinoza’s birth year remains wrong).
Summary [ edit ]
The work is divided into three books, each of which is subdivided into chapters; each chapter generally deals with a single philosopher, school of philosophy, or period of time.
A battery made from zinc and lignin that can be used over 8,000 times has been developed by researchers at Linköping University, Sweden, with a vision to provide a cheap and sustainable battery solution for countries where access to electricity is limited. The study has been published in the journal Energy & Environmental Materials.