Entropic resonance and the completion of quantum gravity
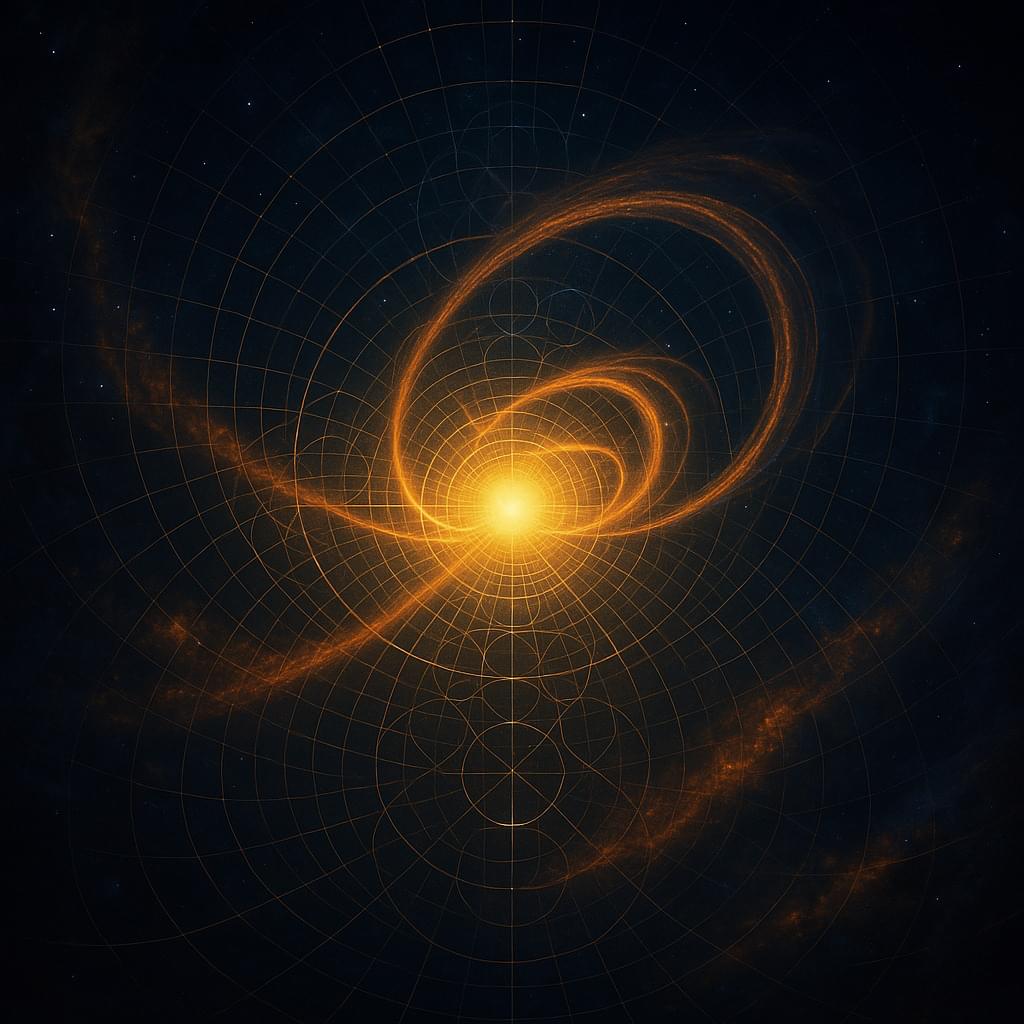
npj Quantum Inf ormation volume 11, Article number: 37 (2025) Cite this article.
Magnesium is a common chemical element, an alkaline earth metal, which is highly chemically reactive and is very light (even lighter than aluminum). Magnesium is abundant in plants and minerals and plays a role in human physiology and metabolism. In the cosmos, it is produced by large aging stars.
Among its physical properties, while it is a good conductor of electricity, magnesium is not known to be a superconductor. Superconductors are particularly promising materials with the potential to revolutionize energy transmission, medical imaging, and quantum computing, and are defined by their ability to conduct electricity without resistance below a certain critical temperature.
Recently, with my colleague Giovanni Ummarino from Turin Polytechnic, I have started challenging the textbook paradigm that states only certain elements in the periodic table can be superconductors. In particular, my colleague and I have shown that the phenomenon of quantum confinement can turn non-superconducting elements into superconductors. Our research is published in Condensed Matter.
In their ongoing efforts to push the boundaries of quantum possibilities, physicists at WashU have created a new type of “time crystal,” a novel phase of matter that defies common perceptions of motion and time. The WashU research team includes Kater Murch, the Charles M. Hohenberg Professor of Physics Assistant Professor, Chong Zu, assistant professor of physics, and Zu’s graduate students Guanghui He, Ruotian “Reginald” Gong, Changyu Yao, and Zhongyuan Liu. Other authors are Bingtian Ye from MIT and Harvard’s Norman Yao.
Until now, creating quantum superpositions of ultra-cold atoms has been a real headache, too slow to be realistic in the laboratory. Researchers at the University of Liège have now developed an innovative new approach combining geometry and “quantum control,” which drastically speeds up the process, paving the way for practical applications in quantum technologies.
The paper is published in the journal Physical Review A.
Imagine being in a supermarket with a cart filled to the brim. The challenge: get to the checkout before the others, without dropping your products on the corners. The solution? Choose a route with as few corners as possible to go faster without slowing down. That’s exactly what Simon Dengis, a doctoral student at the University of Liège, has managed to do, but in the world of quantum physics.
The effects of quantum mechanics—the laws of physics that apply at exceedingly small scales—are extremely sensitive to disturbances. This is why quantum computers must be held at temperatures colder than outer space, and only very, very small objects, such as atoms and molecules, generally display quantum properties.
By quantum standards, biological systems are quite hostile environments: they’re warm and chaotic, and even their fundamental components—such as cells—are considered very large.
But a group of theoretical and experimental researchers has discovered a distinctly quantum effect in biology that survives these difficult conditions and may also present a way for the brain to protect itself from degenerative diseases like Alzheimer’s.
Such findings wouldn’t have been possible using the traditional resistivity approach. “We demonstrate that the magneto-thermopower detection of fractional quantum Hall states is more sensitive than resistivity measurements,” the researchers note.
“Overall, our findings reveal the unique capabilities of thermopower measurements, introducing a new platform for experimental and theoretical investigations of correlated and topological states in graphene systems, including moiré materials,” Ghahari concluded.
Hopefully, these findings will help us realize the true potential of the FQH effect. However, whether the same approach could be used to detect other exotic quantum states remains to be explored through further research.