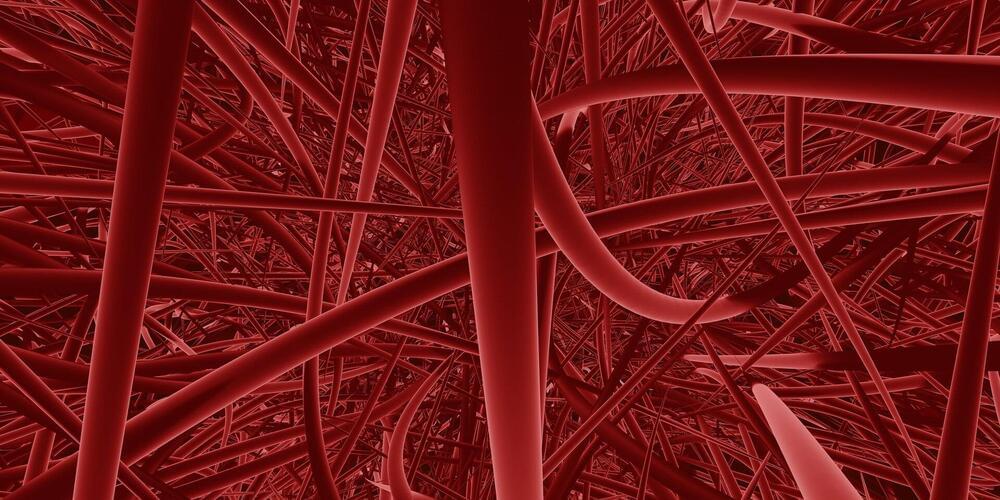
Biological computing machines, such as micro and nano-implants that can collect important information inside the human body, are transforming medicine. Yet, networking them for communication has proven challenging. Now, a global team, including EPFL researchers, has developed a protocol that enables a molecular network with multiple transmitters.
First, there was the Internet of Things (IoT) and now, at the interface of computer science and biology, the Internet of Bio-Nano Things (IoBNT) promises to revolutionize medicine and health care. The IoBNT refers to biosensors that collect and process data, nano-scale Labs-on-a-Chip that run medical tests inside the body, the use of bacteria to design biological nano-machines that can detect pathogens, and nano-robots that swim through the bloodstream to perform targeted drug delivery and treatment.
“Overall, this is a very, very exciting research field,” explained Assistant Professor Haitham Al Hassanieh, head of the Laboratory of Sensing and Networking Systems in EPFL’s School of Computer and Communication Sciences (IC). “With advances in bio-engineering, synthetic biology, and nanotechnology, the idea is that nano-biosensors will revolutionize medicine because they can reach places and do things that current devices or larger implants can’t,” he continued.